Modern plate tectonics is largely driven by slab-pull: a consequence of high-pressure, low-temperature metamorphism of the oceanic crust far from its origin at an oceanic ridge. As it ages, basaltic crust cools, become increasingly hydrated by hydrothermal circulation of seawater through it and its density increases. That is why the abyssal plains of the ocean floor are so deep relative to the shallower oceanic ridges where it formed. Due to the decrease in the Earth’s internal heat production by decay of radioactive isotopes, once oceanic lithosphere breaks and begins to descend high-P low-T metamorphism transforms the basaltic crust to a denser form: eclogite, in which the dense, anhydrous minerals garnet and sodium-rich pyroxene (omphacite) form. Depending on local heat flow, the entire oceanic slab may then exceed the density of the upper mantle to drag the plate downwards under gravity. Metamorphic reactions of any P-T regime creates minerals less capable of holding water and drive H2O-rich fluids upwards into the overriding lithosphere, thus inducing it to partially melt. Magmas produced by this create volcanism at the surface, either at oceanic island arcs or near to continental margins, depending on the initial position of the plate subduction.
A direct proof of active subduction in the geological record is the presence of eclogite and related blueschists. Such rocks are unknown before 2100 Ma ago (mid-Palaeoproterozoic of the Democratic Republic of Congo) but there are geochemical means of ‘sensing’ plate tectonic control over arc magmatism (See: So, when did plate tectonics start up? February 2016). The relative proportions of rare-earth elements in ancient magmatic rocks that make up the bulk of continental crust once seemed to suggest that plate tectonics started at the end of the Archaean Eon (~2500 Ma). That method, however, was quite crude and has been superseded by looking in great detail at the geochemistry of the Earth’s most durable mineral: zircon (ZrSiO4), which began more than two decades ago. Minute grains of that mineral most famously have pushed back the geological record into what was long believed to be half a billion years with no suggestion of a history: the Hadean. Zircon grains extracted from a variety of ancient sediments have yielded U-Pb ages of their crystallisation from igneous magma that extend back 4.4 billion years (Ga) (see: Pushing back the “vestige of a beginning”;January 2001).
Though simple in their basic chemical formula, zircons sponge-up a large range of other trace elements from their parent magma. So, in a sense, each tiny grain is a capsule of their geochemical environment at the time they crystallised. In 2020 Australian geochemists presented the trace-element geochemistry of 32 zircons extracted from a 3.3 Ga old sedimentary conglomerate in the Jack Hills of Western Australia, which lie within an ancient continental nucleus or craton. They concluded that those zircons mainly reveal that they formed in andesitic magmas, little different from the volcanic rocks that are erupted today above subduction zones. From those data it might seem that some form of plate tectonics has been present since shortly after the Earth’s formation. Oxygen-isotope data from zircons are useful in checking whether zircons had formed in magmas derived directly from partial melting of mantle rocks or by recycling of crustal magmatic rocks through subduction. Such a study in 2012 (see: Charting the growth of continental crust; March 2012) that used a very much larger number of detrital zircon grains from Australia, Eurasia, North America, and South America seemed, in retrospect, to contradict a subduction-since-the-start view of Earth dynamics and crust formation. Instead it suggested that recycling of crust, and thus plate-tectonic subduction, first showed itself in zircon geochemistry at about 3 Ga ago.
Detailed chemical and isotopic analysis of zircons using a variety of instruments has steadily become faster and cheaper. Actually finding the grains is much easier than doing interesting things with them. It is a matter of crushing the host rock to ‘liberate’ the grains. Sedimentary hosts that have not been strongly metamorphosed are much more tractable than igneous rocks. Being denser than quartz, the dominant sedimentary mineral, zircon can be separated from it along with other dense, trace minerals, and from them in turn by various methods based on magnetic and electrical properties. Zircons can then be picked out manually because of their distinctive colours and shapes. A tedious process, but there are now several thousand fully analysed zircons aged between 3.0 to 4.4 Ga, from eleven cratons that underpin Australia, North America, India, Greenland and southern Africa. The latest come from a sandstone bed laid down about 3.31 Ga ago in the Barberton area of South Africa (Drabon, N. et al. 2022. Destabilization of Long‐Lived Hadean Protocrust and the Onset of Pervasive Hydrous Melting at 3.8 Ga. AGU Advances, v. 3, article e2021AV000520; DOI: 10.1029/2021AV000520). The authors measured lutetium (Lu), hafnium (Hf) and oxygen isotopes, and concentrations of a suite of trace element in 329 zircons from Barberton dated between 3.3 to 4.15 Ga.
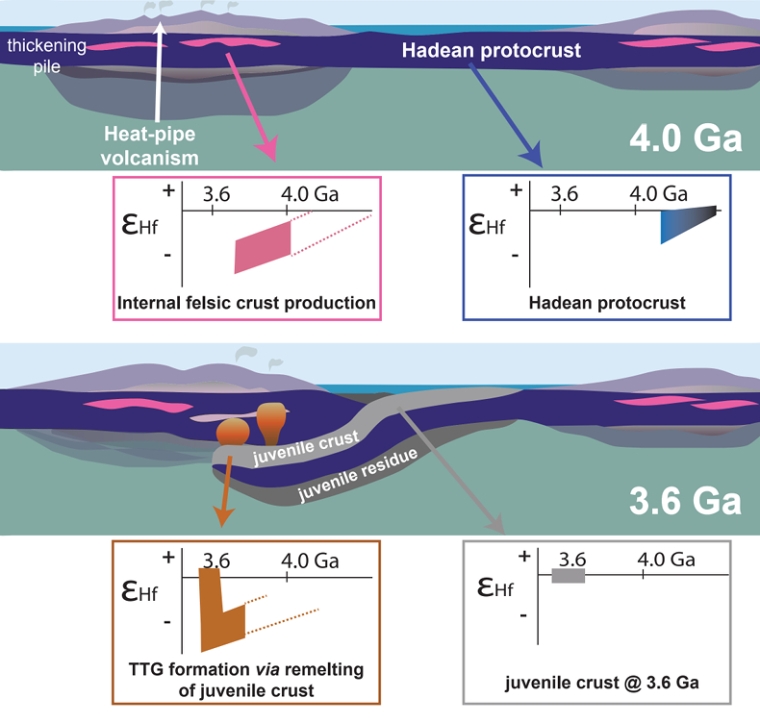
The Hf isotopes show two main groups relative to the values for chondritic meteorites (assumed to reflect the composition of the bulk Earth). Zircons dated between 3.8 and 4.15 Ga all show values below that expected for the whole Earth. Those between 3.3 and 3.8 Ga show a broader range of values that extend above chondritic levels. The transition in data at around 3.8 Ga is also present in age plots of uranium relative to niobium and scandium relative to ytterbium, and to a lesser extent in the oxygen isotope data. On the basis of these data, something fundamentally changed in the way the Earth worked at around 3.8 Ga. Nadja Drabon and colleagues ascribe the chemical features of Hadean and Eoarchaean zircons to an early protocrust formed by melting of chemically undepleted mantle. This gradually built up and remained more or less stable for more than 600 Ma, without being substantially remelted through recycling back to mantle depths. After 3.8 billion years ago, geochemical signatures of the zircons start showing similarities to those of zircons derived from modern subduction zones. Hf isotopes and trace-element geochemistry in 3.6 to 3.8 Ga-old detrital zircons from other cratons are consistent with a 200 Ma transition from ‘lid’ tectonics (see: Lid tectonics on Earth; December 2017) to the familiar tectonics of rigid plates whose basalt-capped lithosphere ultimately returns to the mantle to be involved in formation of new magmas from which continental crust stems. Parts of plates bolstered by this new, low density crust largely remain at the surface.
While Drabon et al. do provide new data from South Africa’s Kaapvaal craton, their conclusions are similar to earlier work by other geochemists based on data from other area (e.g. Bauer, A.M. et al. 2020. Hafnium isotopes in zircons document the gradual onset of mobile-lid tectonics. Geochemical Perspectives Letters, v. 14; DOI: 10.7185/geochemlet.2015), which the accompanying figure illustrates.
See also: Earliest geochemical evidence of plate tectonics found in 3.8-billion-year-old crystal. Science Daily, 21 April 2022. 3.8-Billion-Year-Old Zircons Offer Clues to When Earth’s Plate Tectonics Began. SciNews, 26 April 2022